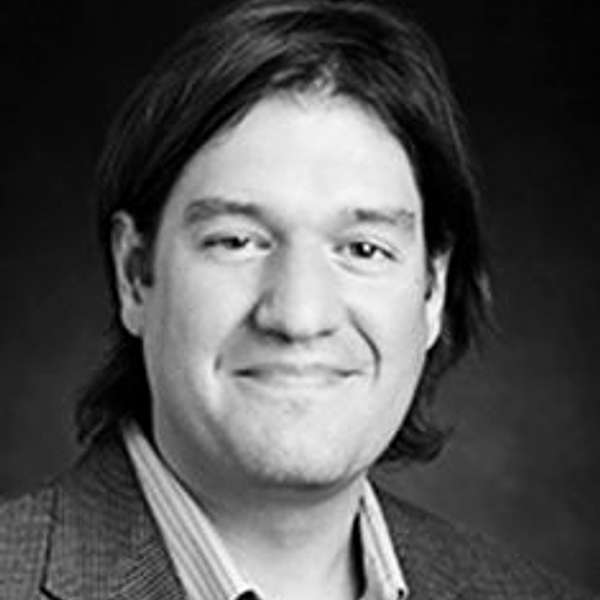
Illuminated: IEEE Photonics Podcast
Illuminated by IEEE Photonics is a podcast series that shines a light on hot topics in photonics, and the subject matter experts advancing technology forward.
Illuminated: IEEE Photonics Podcast
Navigating Future Pathways of LiDAR Technologies
In this episode, Peter Dragic, of the University of Illinois, Urbana - Champaign, shares his expertise bridging a gap between next generation laser sources and active sensing systems such as LIDAR. We'll journey together through the captivating world of LiDAR, shedding light on its applications, complexities, and potential future paths.
Our exploration begins with a look at how this cutting-edge technology is being used in diverse fields such as automotive, surveying, mapping, and archaeology. You’ll gain insights into the challenges of crafting high-power lasers for LiDAR systems, and the pivotal role of laser alignment in ensuring accurate measurements, for example.
Host:
Akhil Kallepalli
Leverhulme Early Career Fellow
University of Glasgow, UK
Moderator:
Rajesh Menon
USTAR Professor
University of Utah
Expert:
Peter Dragic
Associate Professor
University of Illinois, Urbana - Champaign
Have a topic you're interested in hearing about? Let us know!
are finalized, but we'll send you the episode as soon as it's edited, just so you can review it and kind of give it the final approval. I don't imagine you guys are saying anything. We shouldn't, but just in case you wanted to listen really quickly and spot-track it, or you listen to it.
Speaker 2:You mean you'll give us the veto power if we say something controversial.
Speaker 1:Yeah, we actually can. We want you guys to be proud. Like you said, you wanted to put it on your social media. We definitely want you encourage you to be proud of the episode and our editor is really good. So if we want to change anything, we can. The only thing we can't change is the intro song, which is very fun. You guys will hear that. But, yeah, we can edit anything that happens in the episode which goes into what I'm going to say next.
Speaker 1:This is really relaxed. If you start to answer a question or start to ask a question and you feel like you kind of misspoke or didn't say it how you wanted it to come out, feel free to say I'm just going to do that again and we'll edit it out. We're not on live TV, nothing like that. So this is really relaxed and the editor has a really good eye to fix anything if something doesn't flow right. So if there's no other questions, I will pass it off to a kill the host to kick it off, and, feel free, I'll be on with you guys the whole time. So if I hear something that I might say, oh, let's redo that, I might pop in, but likely not, because we do want it to be organic, but if you have any questions that arise during the conversation, I'll be here, or any technical questions.
Speaker 3:All right, excellent, peter Rajesh ready.
Speaker 4:Yep sounds pretty good Excellent.
Speaker 3:So in three, two, one. Hi everyone and welcome to today's episode of Illuminated. I am Akhil and, as the Associate Vice President of the Young Professionals and the Chair of the Young Professional Advisory Committee, it's my pleasure to be your host today. I'm a biomedical physicist myself working at the University of Glasgow as a leading human early career fellow. In my role for the Ixative Ethnic Society, I'm supporting and promoting initiatives very much like this podcast, to raise the profile of valuable young professionals within various sectors. The Young Professionals Initiative is for graduate students, post-doctoral candidates and early career professionals up to 15 years post their first degree. This affinity group within the Ixative Ethnic Society is committed to helping one pursue a career in photonics. We're here to one help evaluate your career goals, to better understand technical pathways and subject matters, refine skills and finally grow professional networks through mentorship.
Speaker 3:Now onto the more exciting our podcast for today. Today is a particularly interesting topic that we're talking about. Working in classical and quantum optics myself, and with a keen interest in time of light imaging and sensing, our topic is of a particularly personal interest to me. We're discussing LiDAR systems, technologies and current questions regarding the state of the field and the future of the field itself. Our very special guest, professor Pete Dragitch, and moderator Rajesh Menon, are here with us. We're going to technology, career journeys, advice from within and beyond research. Now, firstly, thank you very much for listening to this episode. I am already certain it's going to be a great one, and I hand over to Rajesh for the next introduction.
Speaker 2:Great Well, thank you, O'Kill. I'm very excited to be here with both of you. We are honored to have Pete Dragitch as our expert for today.
Speaker 3:Sorry, I know Kristin's already listening in. Could I just go back? Until? When I actually finished about thank you for listening to this episode, I realized if I just scroll the page, I'm doing the introduction for Rajesh as well. I'm going to do the introduction for Rajesh and then I'll hand over to Rajesh again.
Speaker 1:Okay, perfect, good catch.
Speaker 3:Yeah, I mean literally scroll.
Speaker 1:Okay, go ahead, you can just pick up from there.
Speaker 3:Yeah, I'll hand over to Rajesh again in a second.
Speaker 1:Okay, perfect.
Speaker 3:All right, three, two, one. I will now hand over to Rajesh for the episode and for conversations with Professor Dragitch. Rajesh Menon is an engineer and entrepreneur advancing knowledge at the intersection of optics, nanofabrication and computation. He is a professor in electrical and computer engineering at the University of Utah, a fellow of Optica, sbie and a senior member of our very own IEEE. After receiving his PhD from MIT, he co-founded and led a spin-off company for commercializing maskless lithography. His lab in Utah is exploring a multitude of imaging systems enabled by nanofabrication and computational methods, including miniaturized endomyroscopy for deep brain imaging, ultra lightweight optics for airborne images, computationally enhanced diffractive spectral images and many, many more. Rajesh is also invested in the successful realization of laboratory innovations by entrepreneurship and his funnel for companies. It is a great pleasure now that I hand over to Rajesh for the rest of the episode. Take it away.
Speaker 2:Great. Thank you very much for the kind introduction, Akhil, and it's an honor to be in this fine company and very excited to talk about a very pertinent topic, which is LIDAR. It's the topic of this podcast. Lidar, of course, stands for light detection and ranging. It is a remote sensing technology that uses lasers to measure distances and many more things about the 3D environment which we will learn about today. Most of us have some familiarity with automotive LIDAR. In automotive LIDAR, laser beam is emitted from a source and we measure the time it takes for the beam to bounce back off from objects in the environment. This data is then used to create a detailed 3D point cloud of the area being scanned. What is really exciting now is the creation of 3D point clouds such as automobiles, but including surveying, mapping, forests, urban sprawl, archaeology, environmental monitoring, etc. It's a very exciting technology, but just getting very wide usage, which is, of course, the goal of almost any technology that comes out of the lab. Today we are honored to have an expert in the field of LIDAR, Professor Peter Dragic, with us to geek out about LIDAR.
Speaker 2:Pete received his PhD from the University of Illinois at Urbana, Champagne in 1999. After a stint doing startups, he returned to his alma mater, where he is currently an associate professor in the Department of Electrical and Computer Engineering. His graduate work focused on the development of fiber laser-based sources for atmospheric LIDARs, namely for the sensing of water vapor. His current research interests range widely from optimal materials to lasers and laser systems, including LIDAR. A particular interest are fiber laser systems, which offer some advantages over their counterparts. However, they also suffer more significantly from power limitations due to nonlinearities. Therefore, much of Pete's attention has also been focused on reducing the impact of these nonlinearities, and optical fiber systems for applications such as remote sensing.
Speaker 2:The first of two current LIDAR projects is an upper-demospheric slash exospheric LIDAR for the detection of metastable helium. The other is the sodium LIDAR that will take a ride on a sounding rocket through the lower to middle thermosphere. It is my distinct pleasure to invite Professor Pete Dragic to this conversation. Pete, let us start with an introductory question about could you share with us how you chose to get into the field of LIDAR and what inspired you to pursue these topics? Sure thing, Rajesh.
Speaker 4:Thank you very much for the kind introduction. I'm also very pleased to be here in the company of some really wonderful people, some great minds. To answer your question, I guess this goes back to my undergraduate days. During the end of my junior year I was looking around for a summer undergraduate research experience pretty much of any kind. Frankly, I wasn't sure if graduate school was for me or not, but I was fortunate to find a faculty member working on semiconductor-based LIDARs who was also looking for undergraduate researchers. At the time. His group was trying to develop a system to measure atmospheric water vapor concentrations.
Speaker 4:Water vapor turns out as a very strong and indeed the most abundant greenhouse gas. It helps to trap radiation, keeping thermal energy from escaping the earth's atmosphere. Some of us understand that to be a source of global warming. Anyway, I spent a summer working on the laser transmitter and became passionate about lasers and, frankly, I've been at it ever since. In fact, I became a graduate student in the same group where I did my undergraduate research. I also had a NASA fellowship during my graduate days from the Goddard Space Flight Center, helping with a similar system, actually for the Martian atmosphere. The goal there was to look for terrestrial vents of water vapor that could be indicators of the presence of things like microbial life. What's neat about LIDAR and this is kind of what keeps me going is, if you like lasers, for every LIDAR that you build, you get to build a new kind of a laser system, and so I think that's really really neat and very exciting.
Speaker 2:Wonderful. Thank you, pete. It's quite instructive that you were inspired by an undergraduate research experience, which really underscores the importance of supporting and mentoring students, which I think we'll come back to later in our conversation, and I'm also fascinated by your PhD work about detecting water vapor in the Martian atmosphere. Clearly, it would be one of the greatest discoveries of human history if we were to detect proof of extraterrestrial life forms even outside of solar system. This is something I would like to come back to as we go through this conversation, but let us begin with something more down to earth. Could you give us an overview of what your group is currently working on, the technologies you're developing and their exciting applications that they will enable?
Speaker 4:Sure thing. I'll speak to the two lidars that we're developing in our group, both of which are types of lidars known as resonance fluorescence lidars. So the way that they work is one pixel laser beam tunes its wavelength to an absorption feature of a species. In the case of our systems they are helium and sodium. Those end up being actually in the upper atmosphere. There's a layer of sodium, roughly 90 to 130 kilometers up, that resides there due to ablation of mainly meteorites as they come into the earth's atmosphere. And then helium, in the upper atmosphere ranging from about 300 kilometers to about 900 kilometers. There's a layer of helium, Of course. What can imagine if the atmosphere stratified? The lightest element will appear at the top. And so what we do there? Basically, the principle behind resonance fluorescence is, again, we tune our laser wavelength to an absorption line, like the D2 line of sodium, for instance, at 589 nanometers. That species will absorb that photon and then re-radiate it. It'll re-radiate it as fluorescence and therefore it goes in all four pi-stradians, all directions basically, and we're looking from somewhere like the ground or from somewhere where we have a telescope, the helium lidar. The goal is to measure out to roughly 900 kilometers, 1,000 kilometers metastable helium. So what happens there is that solar radiation comes in and that energetic particles are created, collide with the helium and excite those heliums into an upper state, Many electron volts above the ground state. Once you're up there, there's a feature, an absorption feature at roughly 1 microns 1083 nanometers actually that can be used to probe the presence of that metastable helium in that part of the atmosphere, and so of course we want to make sure our laser wavelength is at 1083 nanometers. What's interesting about this is that's a wavelength that's achievable through fiber laser technologies, among other solid state methods, and what we're interested in doing is creating a high power source that can go up far enough into the atmosphere. Again, it's a resonance fluorescent system. So then that laser signal will be absorbed, It'll be re-radiated to the ground and we sit on the ground with a very, very large telescope, such as an observatory telescope or something like this, and receive signal back Again. If we do, if we pulse the laser or we can actually do other techniques to sort of do range resolution, and we can track and we can watch the helium, that metastable helium concentration, as a function of time and distance, and that gives us information about the dynamics of the atmosphere. So the helium itself serves as a tracer. It's not necessarily that we care to first order about the helium itself, although there are interesting things about that too. It serves as a tracer to give us details about the atmospheric dynamics.
Speaker 4:That second system is a sodium-based system, Again at 589 nanometers. That one is not directly producible by a fiber, but again you start with some sources and you get to a wavelength somewhere in the infrared and you do frequency doubling and there's lots of neat ways to achieve that wavelength. But anyway, the idea is there is a layer of sodium roughly 90 to 130 kilometers, and watching how that sodium moves through the atmosphere gives us information about atmospheric dynamics, the motion of energy from lower parts of the atmosphere to the upper parts of the atmosphere. How do they couple? A lot of that is still not understood by humanity, and understanding the way that functions can give us better insight into creating global energy transport models for our planet. The idea there, of course, the system itself will take a ride on a rocket. What we're looking for in particular for that system is kind of a short-range, ranging from the rocket, say, a couple to a few kilometers, and we're looking for turbulence effects, mixing effects. So what happens during mixing events. We're simply using the sodium, again as a means to watch that process. It's not so much that we care how much sodium there is. Again, there are reasons why you'd want to know how much sodium there is. For instance, there are guide star applications where understanding the concentration is very important, but we're using that as a tracer to actually watch the motion of the atmosphere.
Speaker 2:It's very interesting. I just want to make sure I understand it. In the case of the resonance fluorescence, you are exciting the resonance and watching the fluorescence from the ground. How is the excitation beam scanned across some field of view? How does it actually work?
Speaker 4:We can scan the beam. Most generally, though, we don't scan the beam. It's kind of a line of sight. We look in one direction. The beam will expand to some extent, of course. Think about a milliradian beam going hundreds of kilometers. It'll be a relatively large beam, so we're really integrating across its area. Certainly we can think about a scan. That becomes a little more difficult as one goes to large scan angles because the distance to the measure end is a little bit increased.
Speaker 2:In that respect, how do you actually get the range information from where the fluorescence came from?
Speaker 4:For a LiDAR. You can do this a couple of different ways. The most common approach is to use a pulsed LiDAR, which you alluded to earlier in the discussion today. What you do is you send out a pulse of light. You're basically measuring returns as a function of time. It's a time of flight thing. If you get to some point in time where you start measuring information, you could turn that into or start measuring returns. You could create a distance distribution by scaling via the speed of light. Understanding there's a round trip involved. One of the caveats there, of course, is if you do a pulse system, you want to make sure that your pulse goes out in a way that you don't alias your return signal from the atmosphere. That means that if you send your pulse out, you want to make sure your pulse goes out and then it leaves the line of sight to make sure that you're not getting returns from different parts of the atmosphere, from different pulses.
Speaker 2:Ah, yes, yes, I see that was actually one of my questions was would there be potential for ambiguity if sodium at different distances across the layer starts fluorescing from the same pulse? Is that very possible?
Speaker 4:Yes, it's possible, but because the pulse is propagating at the speed of light through that range, when we receive those returns will be a function of time as well.
Speaker 2:So if we send out a pulse.
Speaker 4:Let's say there are two ranges, X1 and X2,. Well, distance equals rate, times, time via the speed of light, of course, and so when we start receiving signals from the first part, from X1, will be a time different from X2, and that's how we can resolve them. But if we have multiple laser pulses that exist at the same time, then it becomes ambiguous to you know which pulse contributed from which layer.
Speaker 2:Yeah, yeah, yeah, yeah. Very cool, and I would assume that the signal that you receive back is quite weak. Can you comment on that? Like, how much? What's the efficiency from the excitation to the received signal? And if that is a challenge, how do you deal with it?
Speaker 4:Yes, that is a challenge actually. You know you send out a signal. That might be, you know, for our helium LiDAR we're actually envisioning kilowatt lasers. You know the return is going to be many, many, many orders of magnitude below what you put out. Oftentimes it requires photon counting and so you'll actually have a receiver or a detector like a photomultiplier tube or an avalanche photodiode or something that's configured to count photons.
Speaker 4:Of course that depends on the number of returns.
Speaker 4:You know, if you have a larger amount of returns, you want to make sure that your photon counter can keep up with the number of photons that are coming back.
Speaker 4:If your returns are very small or very low the number of photons, then we have to consider, of course, ultimately scaling the receiver design.
Speaker 4:So you know, if our returns are low, you know a couple of photons such that they're swamped out by the noise that we might get, you know, say, thermal noise or background light from some other source, then we think about scaling the telescope, you know. So it's kind of like the trick in LiDAR is power and aperture. So the more power you have, of course, the more returns you're going to get, the more aperture you have, the more of those photons you can collect, you have a larger light bucket. There is, though, a limit to that, especially in terms of the residence fluorescence LiDARs. The residence fluorescence LiDARs, actually, I mean, they're like it's almost like a fluorescent material in space, kind of like a laser, for the most part kind of up in the atmosphere, and so you know, if you put out too much of the laser signal, hoping to get returns back, you can actually saturate the layer, and so there's going to be a limitation, potentially, to how much signal you can get back, regardless of the size of the telescope.
Speaker 2:Wow, yeah, I was just noticing how many different parts of electrical engineering come into play to make the LiDAR work, everything from optics to signal processing.
Speaker 4:Yes.
Speaker 2:I'm curious if I were to dig in a little deeper into the laser itself. You mentioned very high kilowatt lasers. Could you talk a little bit about the challenges and complexity of building such lasers?
Speaker 4:Oh, certainly. Of course, the laser itself is comprised of many components. There's different ways to achieve laser technology. One it could be based on it could be a traditional laser that's based on a resonator, and, you know, you kind of achieve high power that way. Other approaches are to create these master oscillator power amplifier type lasers, where you start with a seed laser, perhaps a relatively low power, and then you amplify it. In fact, both of the systems that we're building are based on that technology. The helium LiDAR is nothing more than a semiconductor laser that's amplified by a chain of fiber amplifiers, and then our sodium system is based on Raman scattering in bulk crystals. In that case, again, it's amplifiers that are amplifying our signal, and then at the end we double to get the 589 from 1178 nanometers.
Speaker 4:To get to the high power, there are several things that of course we have to think about. One is you have to energize, or provide energy to the laser. So the pumps, as we call them, have to provide sufficient power in a wavelength range that the gain material can absorb. That's basic lasers 101. And then you have to consider the modality or the framework by which you're actually generating the light. So, for instance, in fiber laser systems we're after the high power, and for our helium system we want to make sure that our laser wavelength not just the wavelength but the spectrum of that laser lies within the absorption band of the helium. Turns out that in the upper atmosphere that's on the order of something like a gigahertz. This is all a temperature broadened stuff, but anyway we want to make sure that our laser signal is the spectral bandwidth is much less than the one gigahertz. Turns out that in optical fibers this leads to nonlinearities, in particular stimulated brillo on scattering, which is an interaction between the light wave and an acoustic wave within the fiber. That can greatly limit the amount of power that you get out of it. So it's not so much that you want to get the power out of it, but you have to then devise an optical fiber that can also support that kind of power levels while somehow circumventing the effects of brillo on scattering.
Speaker 4:There are several ways to do it. It might be beyond the scope of today's discussion, and then on top of that, of course, are all the optics, and so normally when we send a beam out, if we're going over long distances it's different of over short distances, but maybe we don't want the divergence to be too large, because as we come up through the atmosphere we don't want to have our beam expanding to many hundreds of kilometers in the far field, and so what we'll do is to reduce the divergence. We'll do a beam expansion and of course all of the optical components that go into that expansion then have to tolerate that kind of power. So there are a lot of different things that we think about. And then ultimately, as we're running, we think about device longevity.
Speaker 4:Are there components to that system that might fail over time, especially when you're doing something like a remote measurement, or you're sending a laser system onto an autonomous vehicle or even a rocket, because I wouldn't call that necessary. It's autonomous, I guess, in a way, but if you're just launching and it's doing its thing, you want the system to succeed and not fail during its flight. And then, on top of that too, of course, when you're on a platform such as that one, you think about vibrations, things shaking. What's interesting about that system is it's not so much making the measurement once we get to the layer that we're interested in the sodium layer, it's the ride that it takes getting up there, and so when things are shaking, it has to survive the ride.
Speaker 4:Once it gets to the layer, everything's nice and smooth, all the rocket engines shut off and it's not a big deal. But then we also think about making sure that the wavelength itself is stable enough again to be held within the absorption band of interest. There are temperature fluctuations, vibrations and things that are happening. There's a possibility of the wavelength walking off For systems with motion, such as the rocket. Now we also have to contend with a Doppler shift. So the fact that our rocket is moving gives us another degree of complexity, in that we have to understand not just the wavelength, but how does the observation change, given the fact that the rocket itself is on a moving platform?
Speaker 2:Wow yeah, there are so many layers of complexity that you just went through that. I want to peel away a little bit and ask you a little bit more detail. The stimulated balloon scattering is an incredibly fascinating phenomenon and I just want to clarify with you. It is the. Where does the acoustic wave arise from? In the fiber. So let's start there. I have so many questions about what you just explained.
Speaker 4:Oh sure, sure. Well, I guess the easiest way to think about it, the simplest model, is to consider the fiber itself as being at temperature, it's just room temperature. Whatever it is, there are vibrations that are present in the material, and so you could think of sort of the glass itself as being kind of a bath of acoustic waves that are all present. And as the light wave passes through the optical fiber, there will be bragg mesh with some of those acoustic waves, as your bragg. The acoustic wave itself, of course, is a longitudinal pressure wave, and if you have pressure in the material, that changes the refractive index, and so the grading itself looks a lot like a fiber bragg rating. And so your optical wave, when it comes in, will be bragg meshed with some of those acoustic waves. Again, one in principle.
Speaker 4:But the optical fiber can be an acoustic wave guide and it can actually interact with multiple acoustic waves and so on and so forth. But to keep it simple, the light wave will then, will scatter from that bragg-matched wave. The scattered light wave will then well, it's also Doppler shifted, by the way, because the sound wave is moving at the speed of sound. So you have a frequency shift associated with that reflected wave. That scattered wave then will interfere with the original forward-going wave. That interference pattern then feeds that original acoustic wave, because now the original acoustic wave becomes amplified, its reflection becomes stronger, and so you can kind of see this positive feedback cycle sort of forming.
Speaker 4:Eventually you reach some power threshold known as the threshold power, I guess where your stimulated process takes over from noise and instead of getting light out of the fiber, much of it gets reflected back towards the center. So it's a problem that you see not only in lasers but also in telecommunications. In telecom the easiest way to avoid this problem is to broaden the spectrum, as Brillouin scattering is a coherent process that requires a line width that's less than the Brillouin line width, which is usually on the order of tens of megahertz. For us, for the LiDAR application. Oftentimes we're not allowed to do that, because we're trying to make sure that our laser signal lies within the absorption bandwidth of the species we're trying to measure.
Speaker 2:I see, wow, very cool. The second topic I was interested in was the. You know, it's pretty incredible that you're working with these huge powers and I'm curious if you can comment on how, practically speaking how, these experiments are done in a university lab. I'm sure there are some interesting challenges there.
Speaker 4:Yeah Well, safety is an utmost concern, so making sure that the students are aware of their goggles and all that stuff. Actually, if I could take a side note before we move on, yes, of course.
Speaker 4:We actually have partners that do that. We partner with Air Force and other groups that do kind of the high power building for us, and so we don't. One of the things that I try to do make sure that you know it's a lot to ask a student to be comfortable about, you know, working with kilowatts, yeah, so certainly underscores the importance of collaboration. Yeah, so this was. This is a side, that was a side comment, not meant for publishing, just just if that's.
Speaker 2:I guess the editors would Sorry, I couldn't find the mute button. Yep, that's fine, peter, okay, okay, yeah.
Speaker 1:I also just wanted to take this opportunity to say that it's going really good. The podcast is so interesting, so I'm really excited about this. Okay, yeah, I don't know if we're going off topic by talking about the FIWAR technology or not?
Speaker 4:No, you guys.
Speaker 1:I mean, like I said, it's a conversation in lead back and it's really interesting Me and Karen are just saying how we're both learning so much, so you're doing great, but I'll just make sure. So you just want to remove from where you said to the side note right, yeah, yeah, yeah, yeah.
Speaker 4:No, I mean like I said, we We'll go up to 100 watts, a couple of 100 watts, but that's sort of where I like to draw a line, because beyond that point there's a real risk for the students and unless they have a lot of experience working with these things, it's better if someone else is willing to do it for us. Yeah, here you go.
Speaker 1:Yeah, yeah no, that makes complete sense. Okay thank you so much. I took no, okay. Okay, I'm going to go back on me.
Speaker 2:The other question I had was relevant to the rocket launching, so can you talk a little bit more about the challenges involved in designing a laser to be launched on a rocket Sounds incredible. Yeah, well, we have.
Speaker 4:The R LiDAR system is comprised of many parts, as we've already talked about. You know there's the. I guess the rocket is fairly long and thin and when it flies it has to fly in a way that's stable and, I guess, predictable. But there are multiple components that we have to worry about. So one, there is a telescope that's on board, you know, a curved mirror that's on board the rocket, and so you know when the Actually the way it's designed is as the rocket goes up through the atmosphere, you know different. You know the fuel pods or whatever will fall off the back end. I'm not an expert in the rocket itself, by the way, but thinking about you know kind of ruggedization. So one, you have to make sure that that telescope or that mirror survives that process. So actually figuring out how to epoxy it to a stable sort of substrate such that it doesn't crack or shift or move out of place, that's really important. Of course the. You know we have our mirror and that's in a different place from the laser. The laser itself is on a hardened breadboard. All the components are in, you know machined grooves, kind of where everything sits, you know mechanically ruggedized and of course, locked into place with various epoxies and then the output of that system, of course. Well, I should go back and comment. One of the things about this setup and you know when one is thinking about making sure that the laser itself stays aligned during that flight is to use components that are a little bit bigger than you would usually use in a lab. You know it's kind of in. You know it's like a typical, you know, university laboratory or something like this teaching lab or research lab or whatnot. So what you want to make sure is we have things like lenses and mirrors that direct different parts of the beam to different locations inside the laser itself If something shifts. You know, like the crystal, for example, the Raman crystal is large enough such that if there's a shift in the position it doesn't matter because the crystal is large enough to tolerate that change in position. So that's part of a key to that. The other part of it, of course, is making sure that things stay put. You know you want to make sure that everything is locked into place.
Speaker 4:We actually I'm not a mechanical engineering expert, but this is a testament to the multi, you know, kind of multifaceted nature of doing research like this is. We have people from the mechanical engineering department helping us to design that stuff. You know, it's very, like I said, very multidisciplinary. It's like, you know, how do we? I understand photons and I understand how to generate them, but how then do we drugadize this thing? Okay, and so that's part of the, you know, yeah, yeah, part of the whole story.
Speaker 4:And then, of course, as our laser is in a different position from the mirror, you know we'll take an. You know, for example, our concept is to bring an optical fiber, you know, to the output of the laser, and then the optical fiber will bring the beam over to the telescope and then our beam will, you know kind of couple to the telescope, be reflected from the mirror and outwards in a truly monostatic configuration. And then that same telescope is used for receiving Another optical fiber on the receiver end of course, collects that light and then takes that to yet another part of the rocket that has our photodetectors, which are actually photomultiplier tubes, and making sure that that stays aligned is also imperative. So it's, you know, there's fibers, there's the mirror, there's the laser, and it's the alignment of the various things, plus the rigidity, the structural integrity to make sure that things don't break apart, shake apart or become misaligned. So there's a lot to think about. It's not a trivial task, it's just, yeah, a lot of things to think about.
Speaker 2:That's quite an understatement. It's very exciting and I assume this is one of the reasons what makes you so passionate about this research, because it's always something new to learn and problems to solve. I'm curious just once the rocket actually reaches the layer that you're interested in, does it simply float? Like are you still controlling the motors? Like how does it actually work?
Speaker 4:It's going to.
Speaker 4:Basically it's going to ride up to roughly 130 kilometers, you know, through its fuel that's going to be on board and once that's spent the rocket will no longer be.
Speaker 4:You know, it won't be propelled, it's going to undergo a controlled fall and the way that we have it set up is it's actually going to be spinning as it's falling. Of course, those are the rocket scientists that figured that out. Again, there's a whole team of people involved in this development. But as it's coming down it's going to kind of rotate and so what we're going to do is we're going to get a kind of a 360 degree field of view of the returns or the turbulence field that's in the vicinity of the rocket. Again, this isn't turbulence caused by the rocket, it's, in principle, turbulence-related atmospheric dynamics and that fall process is actually quite smooth. Actually, the way they describe it is like a turbulence-free kind of floating. That happens, and you know we can only measure, you know, through the sodium layer which ends at about, you know, 80 to 85 kilometers or so, and then the rocket itself will land in the ocean, near the shore, and will retrieve it to hopefully it'll survive, of course, that process, the impact, so that we can use it again.
Speaker 2:That's incredible You'll get your laser back.
Speaker 4:Well, that's the hope. That's the hope you never know.
Speaker 2:So let me ask you about the another challenge that many of the listeners are probably aware of is, since we are in the in LiDAR, since we are trying to measure such a weak signal, can you talk a little bit about the detection challenges on the detector side?
Speaker 4:Certainly. But again, you know, when you're designing a LiDAR system you kind of have to have a priori sort of a sense of the signal that you expect to get back, and that will help to guide your choice of receiver or detector. The first order, of course you know you have a wavelength that you're producing and then some other wavelengths. Maybe the same wavelength or a different wavelength will come back, and you want to make sure that your receiver, of course, spectrally overlaps with what you're trying to detect. So you know, for instance, you know if you're trying to look for a 1300 nanometer signal with a silicon detector, that's not going to work so good. So of course that's the first priority. The second is to understand how much power or how many photons you expect to get back. If you're expecting a large signal to come back, oftentimes if you're close range to an object and it's, you know, perhaps an automotive LiDAR you might get a lot of signal back, and so you can use a standard detector for something like this, whereas if you are expecting to get just a few photons back, then we have to move to a photon counting device. So those could be photomultiplier tubes. Photomultiplier tubes work on the photoelectric effect.
Speaker 4:Basically, you have this material, you know a photon comes in, the energy is above the work function, it spits out an electron and then that's amplified through a chain of what are called dinodes and you know you get, you know, maybe a factor of a million amplification.
Speaker 4:In that case, just like with anything else, the higher the work function, the lower the noise that you have. Same as with semiconductors. You can also think about photon counting semiconductors. However, the smaller the band gap, the more noisy they become, as thermally generated electrons can traverse the band gap. And so oftentimes, when we're dealing with longer wavelengths, you know where, like, the work function is low or the band gap might be low, then we have to think about actively cooling the device. Yeah, fortunately for us with the sodium those wavelengths are long enough, or sorry, the energies are high enough. The wavelengths are short enough that we can get away with an uncooled receiver system, whereas once somebody moves, once you move to like 1083 nanometers, where there could be a lot of background, light background and then thermal noise, of course, in the receiver system, then we might have to think about cooling the device.
Speaker 2:So actually there's a question for you, Kristen how are we doing on time? I just realized we've gone quite a bit long, Do I?
Speaker 1:kind of have to wrap up yeah, go ahead. Yeah, we have 10 minutes left. We don't have to like stick to the 10 minutes, but I definitely recommend not going that much over. It may be just if you want to pick out something that you really wanted to make sure gets included, but I would try to keep it within like 15 minutes if possible.
Speaker 4:Well, do you guys want to? I've got this text written out for, like, what are other types of light ours? Could that be like a good closing thing, or? I mean, I wrote that out Perfect.
Speaker 2:Yeah, I don't know Right there yeah.
Speaker 4:This way I could just read it and then you know we could. That way, we would bring the last 10 minutes.
Speaker 2:So don't we need to talk about Akhil's questions, or no?
Speaker 1:Maybe like it's just Peter's 10 minutes, Sorry yeah yeah, yeah.
Speaker 3:So if Peter and Rajesh could actually finish in the next 10 minutes, then I've got a couple of questions and I'll try and combine some of the questions. So I asked the important ones and overall we'll still stay within the time period.
Speaker 2:So first let's move on to Dr Kamala, Is that okay, Peter?
Speaker 4:That sounds great. Rajesh, do you have it open?
Speaker 2:Yeah, I have it open, oh, okay.
Speaker 4:Yeah, so I was thinking maybe this kind of this what are other types of lidars? Just to kind of talk about other you know, Okay, cool.
Speaker 2:Okay, three, two, one. So, Peter, so far you've given us a fantastic description of the challenges involved in your specific research. I'm curious what are the types of lidars exist? And also if you can also talk a little bit about opportunities and where this field is going to create.
Speaker 4:Sure, that'd be wonderful. Thanks for the opportunity. So other types of lidars well, we've already discussed the residence fluorescent systems. Others are based on either the elastic or any elastic scattering of light from the atmospheric constituents. In the case of elastic scattering, these are based on scattering from molecules or aerosols. Usually we think about Rayleigh or Me scattering in that case. For example, upper atmospheric, such as exo or mesospheric Rayleigh lidars are most often used to track dynamics in those regions of the atmosphere. For example, one such dynamic process involves gravity waves. These are distinct, of course, from gravitational waves. They relate to the motion of the atmosphere up and down vertically with time. Importantly, tracking events such as these things again also helps us understand how energy couples between the regions of our atmosphere and enables global energy transport models. Aerosol lidars can, for example, be also used for tracking air quality near the ground. So pollution sensing and that kind of thing.
Speaker 4:Lidars that make use of Rayleigh or Me scattering also include something that Lidars known as differential absorption Lidars. There what you do basically is you have your laser beam again it's pulsed, most often times to do ranging, and then you tune your laser beams on an absorption feature and off an absorption feature, if you know what the absorption cross-section is for that feature. The ratio of those two signals gives you a relative abundance of that constituent. These types of Lidars can be used to measure greenhouse gases again, such as water vapor, as I had worked on in the past, and carbon dioxide, for instance, as well. These two atmospheric constituents, among others, of course, can be measured in the near infrared. That means that we can use less expensive and possibly less noisy sensors and less expensive lasers, for instance. Other gas sensors, such as those that look for nitrogen or sulfur oxides, require lasers in the mid-infrared, which is more challenging from several perspectives. Different lasers at those wavelengths produce insufficient powers, especially if you want them to be pulsed Detectors. Of course, now you're talking about low-band gap materials, they become more noisy receivers. You also have Doppler systems that make use of Rayleigh scattering, but the receiver is able to measure a change in the frequency or the Doppler shift. These systems are often actually these days commercially used to measure wind speed in the vicinity of wind turbines.
Speaker 4:Other LiDAR technologies based on any elastic scattering are like Raman and Brillouin lasers. Like Doppler LiDARs, these systems rely on the measurement of change of frequency of an outgoing laser signal, of course after interacting with the measure end. For instance, we talked about Brillouin scattering. Brillouin scattering is an interaction between a light wave and an acoustic wave, oftentimes ultrasonic or hypersonic. The light scattered by Brillouin scattering, just like it is an optical fiber, is frequently shifted, and this frequency shift can be a very strong function of environmental things like temperature. Just as a result of this, for example, brillouin scattering based LiDARs have found applications in ocean thermometry, which becomes a very important thing to measure Again global circulation models, not in the upper atmosphere but in our oceans.
Speaker 4:Lidars can also operate in both the time and frequency domain. We talked about pulse LiDARs, but the time domain systems are pulsed Again. These are time of flight systems. Frequency domain LiDARs, on the other hand, use frequency modulated continuous laser, where the laser wavelength is swept in time. There part of the output beam is taken as a reference or a local oscillator. Then think about like you send a signal out. If our frequency is constantly scanning or sweeping, then the time delay between what comes back and the local oscillator will give rise to a frequency offset between those two things. Then, of course, if we beat them together and measure the frequency shift, that gives us the time delay or equivalent of the distance. Lots of different ways to do this. As far as opportunities and bottlenecks and things go.
Speaker 4:Challenges again, there are a lot of challenges in the development of LiDARs. This is related to the laser wavelength and power. Going back to the automotive LiDAR application, ice safety becomes a concern. Ice safety is a very significant, a very serious limitation to the feasibility of LiDAR systems. Again, we want large telescope. Necessarily. We want high power. You can't think about really putting power out that might damage someone's eye. You also can't imagine putting one meter telescope on top of a car. That's one way to think about limitation. Again, low noise, high efficiency receivers can be a challenge.
Speaker 4:We talked about silicon detectors that have been developed for many years, a very mature technology. Dynamic range available from them is also very large. You can do photon counting with silicon all the way up to high power measurements. But if a desired operating wavelength lies outside that spectral response of silicon, different materials have to be used. Again, some of these things aren't then amenable to photon counting. We have to think about different ways to detect these signals, such as a modern push towards things like far infrared, superconducting, single photon receivers. Anyway, a lot of opportunities in new lasers, new receivers, autonomous operation, of course, power, more efficient solutions are important. We think about all those things as well. Again, as we talked about the environment and the platform on which the laser is placed or the LiDAR is put, it's going to be a serious limitation.
Speaker 2:Wonderful. Thank you, pete, so much for spending the time going through lots of the details about not only your project but also an overview of the opportunities and all the excitement around LiDAR technology. I have certainly learned a lot and enjoyed this conversation very much. Now I'd like to pass the baton to Akhil for the remainder of the podcast.
Speaker 3:Thank you very much Rajesh, thank you very much Peter. That was absolutely incredible. The amount of things that we've actually had a conversation about and so much of what we can unpack through the course of the podcast and afterwards is incredible, and I thank you both very, very much for that. I've got a couple of questions before we close for today, and the first question I'd like to keep for a minute Peter, both interested.
Speaker 3:Now, obviously, as I have learned, being a PhD student and an early career researcher and a postdoc, the first thing you do when you start talking to somebody is you Google them and you find out what they actually do. Now, interestingly, with my background in biophotonics, everything that Peter does in terms of time of flight imaging, in terms of time of flight sensing, lidar systems, low light conditions for LiDAR systems specifically, all the scattering methods, everything are also seen in Rajesh's research of brain imaging. Do you ever see the crossover across the fields? Do you think something in LiDAR systems would be interesting to Rajesh and do you think something in biomedical optics would be interesting to Peter? A question to the most of you.
Speaker 2:Peter, you want to.
Speaker 4:That's a very interesting question because although the different fields use different names, different words for technologies and methods, a lot of the approaches are all identical FMCW, for instance, LiDARs, which you find at automotive, LiDARs, automotive, autonomous vehicle sort of things. The same technology can be found in precision metrology on the micro scale, so that could also include bio applications and bio imaging things.
Speaker 3:Because it's quite interesting, when Peter was talking about everything he was mentioning, he was talking about fluorescence, about LiDAR, about valence scattering, all of which I'm sure Rajesh and myself have seen in tissue imaging. So, rajesh, you were about to say something.
Speaker 2:Peter's exactly right. I think there's a significant amount of cross talk and it's one of the reasons I try to actually talk to folks outside of my discipline is that you not only recognize areas that are common but new ways of solving very similar problems. So in dealing with scattering, certainly in macro scale imaging, people have applied TemuFlight for imaging through scattering media in the microscopy community because the dimensions are so small that the time difference of the reflector pulses are way too small, at least as far as I'm aware of. I think it's quite challenging, but I wouldn't say never, but it is a little more challenging than doing it for much larger distances, I would say. But it feels like adaptive optics clearly have been applied in both areas. Biophotonic adaptive optics is pretty standard nowadays, but it was adopted originally from astronomy and I have completely different fields.
Speaker 3:Absolutely. I mean there is obviously. There's a big difference, literally, between a mirror used in the telescope and a mirror used on a digital micrometer device, but we're still effectively still using a mirror.
Speaker 2:Right, right yeah. So, it really underscores the I think it's an important point that you're raising. It underscores the importance of understanding the fundamentals of whatever it is that you're learning, because as an engineer or scientist, it's much easier to translate your skills to very different fields. I think it's an important point to convey to the young engineers and scientists who are listening to this.
Speaker 3:Absolutely. My next question again to the both of you is, as quickly as you can and as easily as you can, what are the two things, one for a academic sphere and one for a personal? Or? Here's a good advice to be a good researcher. Kind of advice would you give to somebody listening to this? Where should the next LiDAR systems engineer focus on? Also, what should every young researcher try to do right now? And maybe, Peter, would you like to go first?
Speaker 4:Ah, boy okay.
Speaker 2:I can go first if you want, I mean.
Speaker 4:Please, maybe you'll give me some ideas.
Speaker 3:It's just a question of all of us being very polite with each other. So, Radis, how about you go first?
Speaker 4:Well, we can edit these things out, right.
Speaker 2:Yeah, exactly, I'd say as a new I don't want to use the word young, because you may not be young in mind, young in a field, person entering a field. I think it's really important to really talk to as many people as possible to understand the landscape and understand the challenges often which are not necessarily obvious or even written down somewhere. So it's really useful to understand the culture and the challenges of a field as much as possible, and the way I think it's an effective way to understand it is, of course, talk to your peers, talk to your mentors, talk to your competing labs, if there are any, go to conferences. Really try to talk to as many people as possible, even if they're not directly related to your field. You learn a lot. This means my personal experience.
Speaker 4:Yeah, I can actually follow up on that. I agree entirely with that remark, Rajesh. You did your undergrad at MIT.
Speaker 2:My graduate work, but go ahead.
Speaker 4:Sorry, your graduate work? Yeah, we can start over. I fully agree with the comments that you made. Those are very important points. I fully support them. I guess you did your graduate degree at MIT. Do you have familiarity with Olin College's user-oriented collaborative design course?
Speaker 2:Unfortunately not, sorry, please go ahead.
Speaker 4:I actually had the privilege of teaching that as a transplant here at the U of I for a few semesters. It was taught within the Art and Design program, and Olin College actually teaches that as a very first semester freshman course to kind of help students understand how to go out and find problems. It's not so much finding a solution to a problem, but it's going out and understanding how to find where the problems exist. What's a problem? A problem is something that could make someone's life better. A problem could be something that helps us understand our environment better, Anywhere where you feel like you might be able to make a positive change for humanity. And unless you're able to understand how to go out and find those problems, it's hard to understand whether the solutions that you're generating have any value or have any meaning. And so I agree the whole idea.
Speaker 4:That course that I was involved with actually got the students up out of the classroom into the field talking to people. Hey, what do you think is important? What could make your life better From the perspective of a LiDAR system? What is it that we need to understand to really get a grasp on the meaning of global warming? For instance, Do we have to measure carbon dioxide? Or is there an atmospheric model somewhere where there's a scientist who's not a laser person who says, hey, if we had this information, this would be transformative, and so the student then can go out find that problem and then speak targeted solutions to solving that problem?
Speaker 3:I think that's a very interesting perspective and interesting advice as well, because somebody I look up to quite a lot, in addition to everything that you've mentioned, has also quite vocally said that sometimes the people that you actually work with are almost as valuable, if not more, than the actual problem that you're trying to solve, and such is the nature of academia, such is the nature of industry, such is the nature of everything that we do today, that the people that we work with add so much more value. So, going back to Rajesh's advice of speaking to more people, building those connections, and combining that with Peter's idea of the here's a problem I would really really like to solve makes up for such a perfect motivational statement. For instance, where anyone taking on a project, a research problem, across any field, really and I think if you'd actually seen me on video while we were having this conversation, listening to the both of you, I was simply going yep, they've answered that. Yep, they've answered that. Yep, they've answered that. So you've left me with almost no questions to ask, which is really really good.
Speaker 3:I'm going to end today by thanking you both for your time. It's been a wonderful conversation. We have heard about LiDAR systems, about how margin atmospheres can be measured and monitored, the value of it for astronomy, looking at fluorescence, looking at low light problems. We touched a little bit on brain imaging, although that's tangentially related. However, as in research, everything is connected. I think one of the biggest takeaways for me was how multidisciplinary and interdisciplinary research today and problems today have become. There's electronics, there's optics, there's rocket science, and I never actually, and never before today, have I realized how much someone like Peter would really like his laser back after it's gone to space and come back. So I think that's quite nice A journey of a laser that's probably traveled more than me.
Speaker 2:I do have one last question for you, Akhil.
Speaker 4:Yeah, go ahead.
Speaker 2:Before we end, what are some key takeaways that we, as more advanced in the careers, should know how we can support early stage researchers like yourself and your peers.
Speaker 3:Okay, so how much time do you have? How could I list, so, I think, the conversations that people can have? There is an innate fear, when it comes to every early career researcher and PhD student, to walk up to an established academic at a conference and go here's a question I have. I read a paper that you published and I think I have a few questions. Would you have an opportunity for a conversation?
Speaker 3:I think the, as in most academics and most sorry, I correct myself most early career researchers are looking for a mentor, somebody who can either be a mentor for a long duration, somebody who's going to who's at the other end of their career to turn and say I will guide you through this procedure, or somebody who can answer the question as best as they can in that moment, effectively helping them along their journey. I think the best contributions I have had from established academics, from their careers to mine, was simply time, just the opportunity to talk to somebody much further along in the journey and for them to effectively tell me I did this, don't do this or I did this. I think you should also do this. I think there's no better support than a good mentor, some good advice and a generous support of nature really, so does that answer your question? I've got a bigger list, though. Excellent.
Speaker 2:Excellent. No, that's a fantastic place. I've got a bigger list that says sorry, go ahead.
Speaker 3:No, no, no, go ahead. I've got a bigger list that's got cameras, lasers, optic systems. I'm going to put Peter Liner systems on it. It's a shopping bag.
Speaker 1:That was so, so good. I think that might don't tell the other participants, but that's like my favorite episode so far. So thank you so much, I think. Karen Karen did you want to ask a question?
Speaker 2:It was my first and I'm hooked already.
Speaker 3:I found myself taking notes and, like I don't have a clue, but this is so interesting I have to write something down.
Speaker 2:but, pete, you had said when they said what got you into this, you said it was something you did in your undergraduate group work or a study group and that took you back there.
Speaker 3:Can I ask whose?
Speaker 2:group that was.
Speaker 4:George Papin, okay.
Speaker 2:I said to Kirsten, I said it has to be one of our guys, because at one point our entire bog was almost from, you know, Urbana Champaign. Oh yeah, we have to know who it was.
Speaker 4:I don't know if George was ever on the bog, but we've got. I mean, ken Chiquette is in the office next to mine.
Speaker 2:He was president at some point, yeah.
Speaker 4:I know George from other things, but like we, I mean you know we oh yeah. Yeah, jim, and yeah, it's interesting, because you know what one regret that I have is that George's advisor was Bahasa. Okay, I've actually never met him.
Speaker 1:Oh man.
Speaker 4:My grand advisor, or whatever you call it.
Speaker 1:Is it Gary Eden? There too?
Speaker 4:Hey, gary is here. Yeah, he's retired recently. He's spending more of his time in Texas these days, kind of in retirement, but yeah, yeah, gary's here and.
Speaker 2:You know that's such a thing. Does everybody from Illinois eventually head to Texas? Because there's?
Speaker 1:a couple of them I know Shu Ling.
Speaker 2:Everybody went to Texas, Yep.
Speaker 4:Yep, yep. So yeah, I don't know what's going on.
Speaker 2:I guess you know they've got their oil field endowments, I guess they're on pump.
Speaker 1:Yeah, that's hysterical. Um, okay, all right, so we're 15 minutes over, so I know how you guys gave so much air free time, so I'm going to wrap it up so you guys can get some time back. But I just want to just seriously tell you guys how grateful and how much we appreciate you participating in this, and we probably will ask for you guys to come back as a moderator or guest speaker. So just keep your eye open and I'll let you guys know as soon as the episode is you know has been edited by our editor, and then I'll let you guys know as soon as it's up on Spotify as well. Excellent.
Speaker 3:Thank you, thank you very much, thank you.
Speaker 4:Thanks everybody. Yeah, Okay, if they need to, you know to dub over anything, because maybe my you know, South Side of Chicago accent was made things unclear.
Speaker 1:But in that case I'll definitely reach out to you guys. But our editor's pretty good and you guys really did like such a good job. You're all naturals.
Speaker 4:Thank you very much.
Speaker 1:Thanks everybody, thank you, bye, bye, bye, bye.
Speaker 2:Bye.